Main Second Level Navigation
2021 Research Excellence Awards Recognize Progress in Gene Regulation and Tissue Engineering
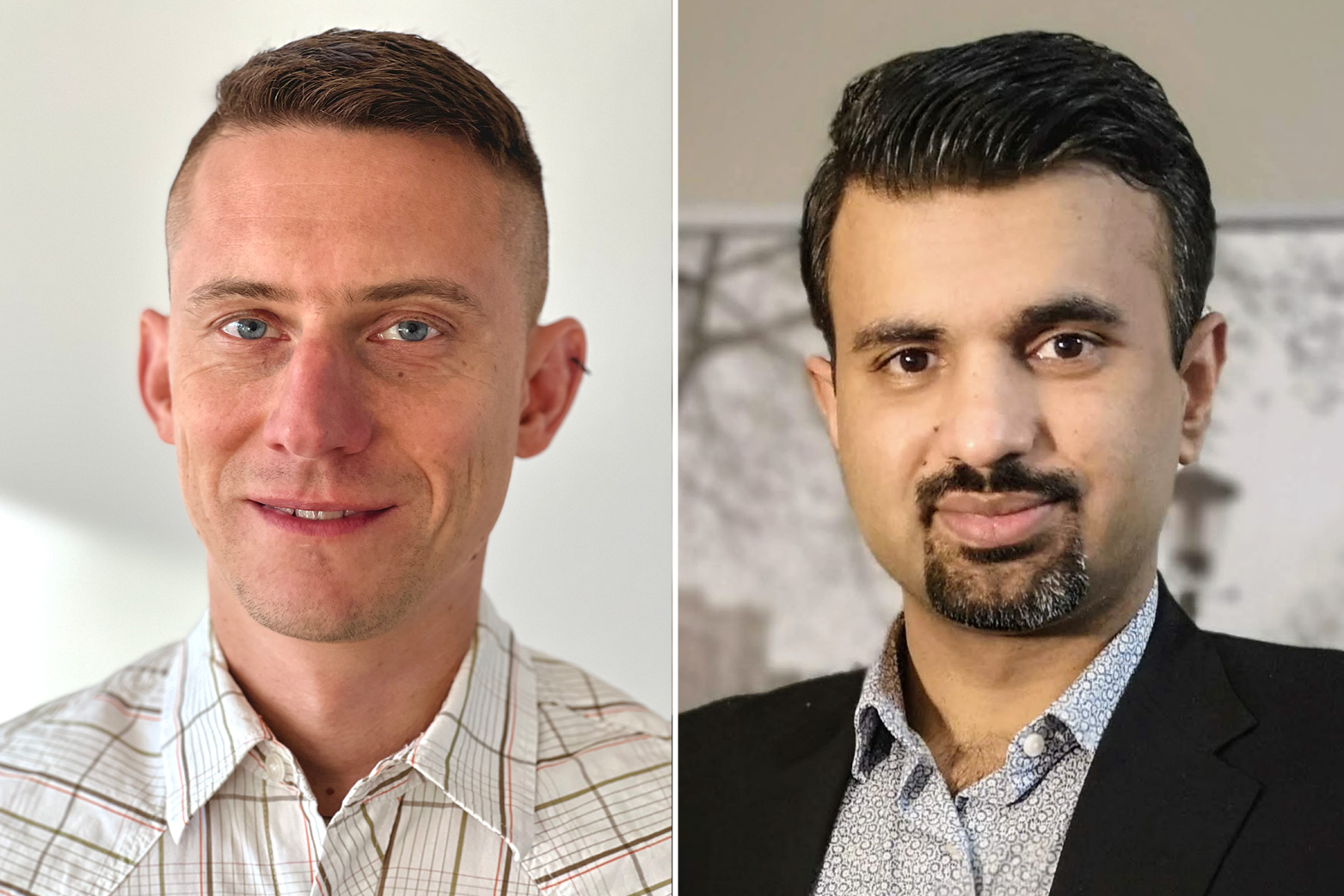
Technological advancement is transforming research and soon it could transform medicine too. Methods developed by two researchers in the Donnelly Centre, Ulrich Braunschweig and Muhammad Rizwan, are driving some of this progress with implications for brain disorders and regenerative medicine, respectively.
Braunschweig is a research associate in Professor Ben Blencowe’s lab in the Centre where he studies gene regulation in the brain and how it varies in syndromes like autism. Rizwan is a research associate in University Professor Molly Shoichet’s lab where he engineers biomaterials that can support growth of liver tissue for research and cell therapy.
The two have now been recognized for their work with 2021 Donnelly Centre Research Excellence Awards. The annual prizes celebrate two postdoctoral researchers in the Centre who are driving collaborative projects at the leading edge of biomedicine.
“I would like to offer my warmest congratulations to Ulrich and Muhammad,” says Charles Boone, a professor of molecular genetics and former interim director of the Centre who helped select the prize winners. “They developed novel technologies in their respective fields which allowed them to ask questions and gain insights that were not possible before. I can hardly wait to see what they will achieve next!"
Ulrich and Muhammad have developed novel technologies in their respective fields which allowed them to ask questions and gain insights that were not possible before. I can hardly wait to see what they will achieve next!Charlie Boone, Professor and former Interim Director of Donnelly Centre
Hunting down genetic miscues in the brain
The brain’s ability to process information is in part borne out by a startling diversity of protein species unrivaled by any other organ in the body. This is thanks to a process known as alternative splicing which allows different proteins to be encoded by the same gene— therefore expanding the molecular toolbox of brain cells—and which has been the focus of Braunschweig’s research.
During alternative splicing, genes’ coding fragments, or exons, are variably spliced into messenger RNA molecules which serve as templates for protein synthesis. Depending on which exon combinations are spliced together, the differences between the ensuing proteins can have a large impact on their function.
The Blencowe lab previously showed that splicing is tightly regulated during brain development and that miscues in this process can lead to neurological disorders such as autism. But many unknowns remain regarding how splicing is regulated in different cell types and at different time points.
Braunschweig sought to shed light this by helping develop a method called SPAR-Seq, for Systematic Parallel Analysis of Endogenous RNA Regulation Coupled to Barcode Sequencing, which allows a systematic identification of cellular factors responsible for diverse splicing events affecting dozens of genes at a time. Among the factors he discovered is a novel splicing regulator dubbed Puf60 which itself undergoes alternative splicing during brain development, which alters its function.
Because SPAR-Seq can be used to study splicing in other cell types and cellular process, Braunschweig established multiple collaborations with other groups in the Centre and beyond to adapt the method for many other research questions.
“The Donnelly Centre is a fantastic, highly collaborative place with opportunities that do not exist elsewhere,” he says. “Whatever you need, whatever you want to know, whichever technology you need, there’s always somebody in the building who can help you.”
In another and unexpected finding, Braunschweig discovered that some splicing errors are more common than previously thought, and that they can even be co-opted by the cells to regulate gene activity. When the genes’ noncoding segments, or introns, are erroneously spliced into RNA transcripts, this prompts transcript degradation to prevent malfunctioning proteins from being made. But in some cases, intron retention serves to ensure that a right protein is made at the right time. Working with John Rinn’s lab at the University of Colorado, Boulder, Braunschweig found that intron retention in a cancer gene called TERT ensures that transcriptsare kept in the cell nucleus where they can't be translated into proteins until a time when the TERT protein is needed at which point the correct splicing kicks back in.
Making unexpected breakthroughs as this is what makes science exciting, but it requires a supportive work environment and Braunschweig credits his supervisor for making this possible.
“Ben is a great scientist and will not rest until we’ve solved a problem. He has a talent to always turn projects into great stories even when they don’t always go according to plan,” he says.
When the coronavirus pandemic hit in March 2020, Braunschweig was quick to join the effort between the Blencowe lab and Jeff Wrana’s lab at the Lunenfeld-Tanenbaum Research Institute, at Sinai Health System, to adapt SPAR-Seq into a more efficient method for detecting Sars-CoV-2 from patient samples. As new virus variants began to emerge, they further adapted the method which has become a key tool for variant detection and surveillance in samples collected at Toronto’s Mount Sinai Hospital. The experience was “eye opening” for Braunschweig and left him with a determination to pursue projects in the future that can have a more immediate impact on people’s lives.
“Being cast into a situation where your work goes beyond blue skies research and has a direct practical impact was new for me and incredibly motivating and rewarding,” he says.
Engineering liver tissue for transplants
About half a billion people globally are living with liver disease and for many of them a liver transplant is the only hope of recovery. But with donor organs in short supply, Rizwan and scientists like him are trying to grow functional liver tissue in the lab that can be used for transplants.
Rizwan’s focus has been on creating bile ducts, tubular structures inside the liver which secrete bile and digestive juices. Diseases affecting bile ducts are the reason behind two thirds of liver transplants each year.
Material scientist by training, Rizwan set out to create a biomaterial that mimics the liver’s natural microenvironment to support bile duct self-assembly from stem cells in a laboratory dish. Stem cells can turn into any other cell type in the body and as such hold potential for growing replacement tissues and organs for regenerative medicine.
In order for stem cells to become the specialized cells that make up bile ducts, they need to be coaxed with a signalling protein called Jagged-1 which is produced by the neighboring cells in a distinct spatial pattern. Jagged-1 activates the Notch signalling pathway which drives cell specialization. The bile duct self-assembly is also enabled by a supporting matrix of proteins and other biomolecules known as Matrigel, which is derived from mouse tumours. Rizwan reasoned that to win regulatory approval for any potential therapy, he would have to develop a method that is free from animal cells and which uses strictly defined components.
To this end, he created a gel-like biomaterial known as hydrogel to which he cross-linked a photoactivatable form of Jagged-1. This allowed him to replicate the pattern of Notch activation in the developing liver on the hydrogel by tracing it with a laser beam. Furthermore, he was able to tweak hydrogel’s viscoelasticity properties to match those of the real human liver which can support bile duct self-assembly without Matrigel.
“We have found that we need synergistic complimentary effect of two things to create bile ducts in a dish,” says Rizwan. “Notch alone is not enough. You need to provide it in a defined three-dimensional viscoelastic environment, and only then will the cells self-assemble into these tubular structures that we call bile ducts.”
Rizwan’s breakthrough not only helps clear the path for regulatory approval of any therapy that might come out of his work, but it has also established the minimal components required for bile duct formation to aid further research into the mechanics of this process and how it goes awry in disease.
Rizwan has been collaborating closely with the group of Gordon Keller, a senior scientist at the Princess Margaret Cancer Centre at the University Health Network, which previously developed a protocol for turning stem cells into the specialized cells that form bile ducts.
“It’s been a fascinating journey since I joined the Donnelly Centre in 2019,” says Rizwan. “I was attracted to the Centre and Molly’s lab by the amazing opportunities to network and collaborate with researchers with various expertise including at UHN.”
“And also by the freedom to do the work that really excites me. If you can convince Molly about the importance of research question and its impact on healthcare, she’ll provide all the resources you need to get it done,” says Rizwan.
Now in the home stretch of his project, Rizwan will soon set off for Michigan Technological University in the U.S. to take up a position as an assistant professor where he will continue to develop engineering solutions for liver disease and other conditions.
“I’m really excited to be working with students in my own lab,” he says.
Follow us on LinkedIn and Twitter to keep up with Donnelly Centre news.
News

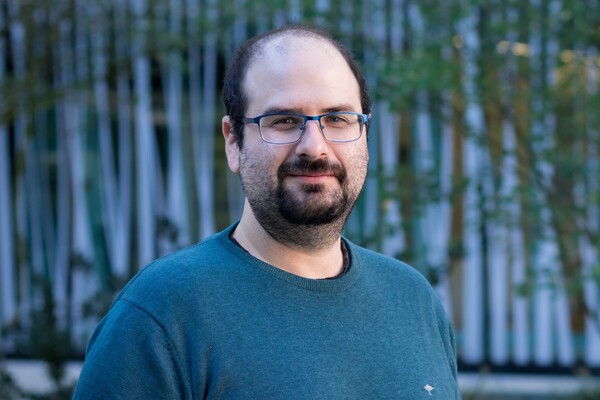